ERC NECTAR
Nuclear rEaCTions At storage Rings
*This work is supported by the European Research Council (ERC) under the European Union’s Horizon 2020 research and innovation programme (ERC-Advanced grant NECTAR, grant agreement No 884715).
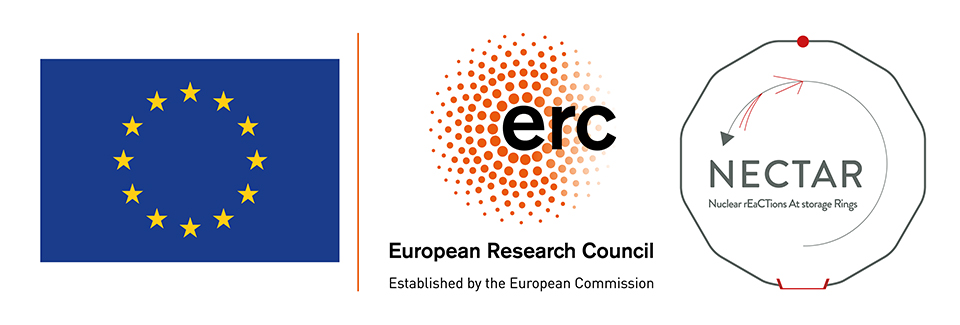
Les sections efficaces des réactions induites par neutron sur des noyaux radioactifs sont essentielles à notre compréhension de l’astrophysique nucléaire et présentent également un intérêt pour les applications industrielles telles que la transmutation des déchets nucléaires. Le projet NECTAR, financé par l’ERC, a pour but de déterminer ces sections efficaces de manière indirecte en utilisant de nouvelles techniques de détection auprès des anneaux de stockage.
Neutron-induced reaction cross sections on radioactive nuclei are essential to our understanding of nuclear astrophysics and also of interest for industrial applications such as the transmutation of nuclear waste products. The ERC-funded NECTAR project aims to determine these cross sections indirectly using novel detection techniques at storage ring facilities.
The NECTAR team at LP2i Bordeaux
Philippe Alfaurt | Control command
Camille Berthelot | PhD Student
Beatriz Jurado | Project principal investigator
Jérome Pibernat | Technical responsible and electronics
Sean PERARD and Mathieu Roche | Mechanics
Remi Faure and Nicolas Tournier | Vacuum
Boguslaw Wloch, Post-Doc
FORMER MEMBERS:
Michele Sguazzin, former PhD Student
Jacobus Swartz, former Post-Doc
Bertrand Thomas, vacuum expert
INTERNSHIPS:
Eliot Guihal, License internship from 2 May to 2 June 2022, supervised by B. Thomas and M. Sguazzin
Camille Berthelot, Master 2 internship from 14 March to 30 June 2022, supervised by B. Jurado.
Damien Sainsimon, License Internship from 2 May to 16 June 2023 supervised by C. Berthelot, B. Jurado and J. Pibernat (a ajouter stp)
Heavy nuclei in the iron to uranium regions are created through processes such as the s- or slow neutron capture and the r- or rapid neutron capture. The latter, which occurs at high neutron densities, is a pathway to highly exotic nuclei through rapid and successive neutron captures, followed by β decays, which may terminate in certain cases in fission reactions. Fission recycling can then occur where the two fission fragments continue to undergo neutron captures and β decays until fission again terminates the r-process path. After a few cycles the elemental abundances can be dominated by the fission-fragment distributions. The knowledge of the neutron-induced reaction cross sections for fission, neutron radiative capture and neutron inelastic scattering for these heavy neutron-rich nuclei is key to our understanding of the astrophysical scenarios that are responsible for the r-process abundance distribution in the solar system.
Apart from their great interest in nuclear astrophysics, the cross sections for neutron-induced reactions also provide valuable information for applications in nuclear technology such as the transmutation of nuclear waste, one of the key problems in nuclear energy to be tackled in this century.
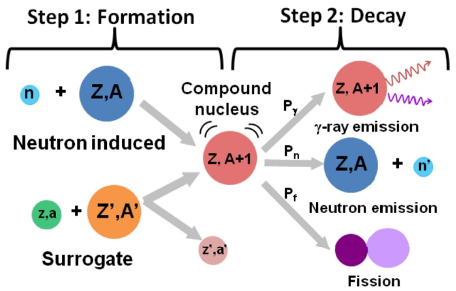
Fig. 1: The sequence of a neutron-induced reaction. First a compound nucleus is formed, which then de-excites by the emission of γ -rays, a neutron or by fission. The probability P associated with each decay channel is indicated. In a surrogate reaction, the same compound nucleus as in the neutron-induced reaction is produced by a different reaction.
To investigate such heavy neutron-rich nuclei, it is not practical to merely bombard them with neutrons in direct kinematics. They are short-lived and typically highly radioactive, which makes safe handling untenable. Additionally, any detection system would be overwhelmed by gamma-ray and particle background, thus rendering neutron capture cross sections impossible to measure in practice. Theoretical predictions of these cross sections can be wrong by several orders of magnitude due to the lack of knowledge on model parameters such as fission barriers, level-densities and γ-ray strength functions, which rule the de-excitation process of the compound nucleus produced after neutron capture.
NECTAR (NuclEar reaCTions At storage Rings) aims to circumvent these problems by using the surrogate reaction method in inverse kinematics. The heavy nucleus is to be put in the beam to bombard a light nucleus in order to produce the compound nucleus that is formed in the neutron-induced reaction of interest by an alternative or surrogate reaction, as illustrated in Fig. 1. Typical surrogate reactions include transfer and inelastic scattering. As illustrated in Step 2 of Fig. 1, the compound nucleus may decay by γ-ray emission, neutron emission or fission. The probabilities as a function of the compound-nucleus excitation energy for these decay modes, which can be measured with the surrogate reaction, are particularly useful to constrain model parameters and to inform more accurate predictions of neutron-induced reaction cross sections.
These experiments are to be performed with the heavy-ion storage rings at GSI/FAIR in Germany. This presents an ideal opportunity for the development of the surrogate reaction method, which is still hindered by unresolved target-related issues. The storage rings at GSI/FAIR provide a high-quality beam which when used in conjunction with an ultra-thin gas-jet target will enable for measurements of excitation energy and decay probabilities with unrivalled accuracies. The simultaneous measurement of fission, neutron and γ-ray emission probabilities will be a first at the GSI/FAIR facility. However, the ultra-high vacuum (UHV) conditions inside the storage rings pose severe constraints to in-ring detection systems.
OBJECTIVE
The objective of NECTAR is to develop a set-up and a methodology to simultaneously measure fission, γ- and neutron-emission probabilities induced by transfer and inelastic scattering reactions in inverse kinematics at the CRYRING storage ring of the GSI/FAIR facility in Darmstadt, Germany.
The required set-up is shown in the upper part of Fig. 2. It consists of: a detector to identify and measure the kinetic energies and angles of the target-like nuclei. A fission detector covering forward angles to detect fission fragments in coincidence with target-like nuclei. A detector for measuring the number of beam-like products formed after g-ray or neutron emission detected in coincidence with target-like nuclei. The detector for beam-like residues is placed after the second dipole magnet downstream from the target. The dipoles will separate the beam and the beam-like residues according to their magnetic rigidity.
The fission detector has to be placed in UHV. This is very challenging and we propose a completely new solution, which is to build this detector with solar cells.
The first experiment will be performed with a 238U beam on a deuterium gas jet target to investigate the reactions 238U(d,p), 238U(d,d‘) and 238U(d,t). This experiment will constitute the final validation of the experimental setup and method. Subsequent experiments will investigate neutron-induced capture cross sections via the surrogate reaction method in multiple unstable heavy nuclei.
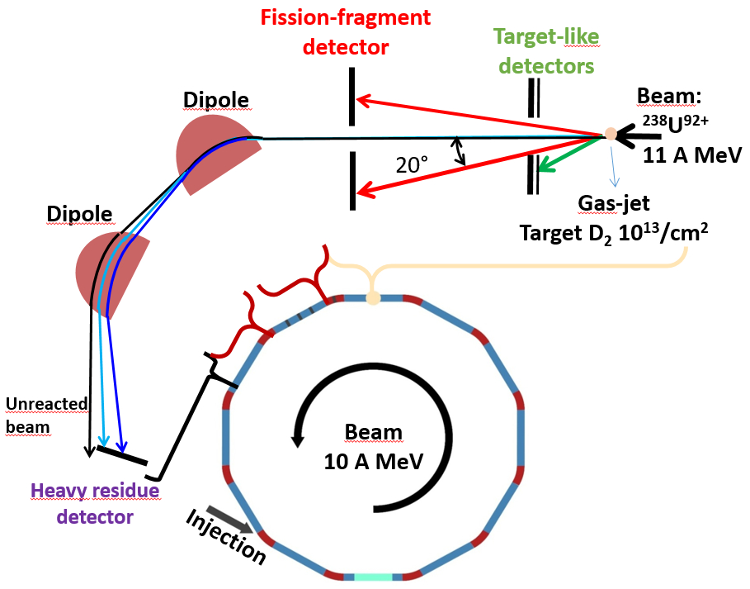
Fig. 2: Diagram of the future NECTAR setup at the CRYRING storage ring facility at GSI indicating the positions of the target residue, fission fragment and heavy residue detectors.
R&D ON SOLAR CELLS
Solar cells, the devices that are routinely used to convert sunlight into electricity, have been shown to have good energy and time resolution when used for detecting heavy ions with energies of about 1 A MeV. In addition, they are extremely cost effective and much more resistant to radiation damage than the traditionally-used silicon detectors. Their radiation hardness will be of critical importance in the ultra-high vacuum (UHV) environment of the storage rings at GSI. Under these conditions, breaking the vacuum for detector troubleshooting during the beam period could cause a fatal loss of time for data taking, since reestablishing UHV, even in a small portion of the ring, can take several days or even weeks.
To use solar cells in our measurements, it is necessary to investigate their response to heavy ions at energies above 1 AMeV and their UHV compatibility.
Measurements were performed at the GANIL in Caen in March 2021 with an 84Kr beam at energies of 5, 10, 15 AMeV on Si and Ge solar cells with dimensions varying from 10×10 mm2 to 30×30 mm2. With these tests we could investigate the evolution of the solar cell response with beam energy and also perform long radiation resistance tests. Preliminary analysis of these data indicates a non-linear response in the amplitude of the energy output signal at the highest beam energies, which is presently under further investigation. These measurements also afforded us with the possibility to test a new preamp developed at CENBG specifically for use with solar cell detectors as part of the NECTAR project. Finally, the radiation resistance tests demonstrated a superior performance of solar cells with respect to silicon detectors.
These measurements were performed to follow up on previous measurements which were made in 2018 and 2019 on solar cells with beams of 84Kr and 129Xe at 7-13 AMeV (A. Henriques et al, Nuclear Inst. and Methods in Physics Research, A 969 (2020) 163941).
In parallel to the studies on the solar cells’ response, we have developed a test bench (TREVO) to investigate the ultra-high vacuum compatibility of the fission detector. This test bench should be operational in 2021.
FIRST EXPERIMENT AT THE ESR STORAGE RING
From the 20 to 27th June 2022 we successfully conducted the first NECTAR experiment at the ESR storage ring.
In this experiment a 208Pb beam at 30.77 A MeV interacted elastically and inelastically with a gas-jet target of hydrogen. Inelastic reactions led to the excitation of the 208Pb projectiles. We measured the inelastically scattered protons with a Si telescope and the beam residues produced after deexcitation of 208Pb via gamma-rays and neutron emission with a position sensitive Si strip detector placed behind one of the magnetic dipoles of the ring, see figure 3.
These detection systems were designed and developed by the NECTAR team. The detector telescope for target-like residues consisted of a 500 µm-thick DSSD followed by six 1.5 mm layers of Si detectors, see left part of Fig. 4. The detector pocket for this telescope, illustrated on the right part of Fig. 4, was designed and manufactured by the Max Planck Institute for Nuclear Physics in Heidelberg with a 25 µm-thick front-window which the target residues traversed to reach the detector telescope. The pocket itself was held at atmospheric pressure within and surrounded by the UHV of the target chamber.
The beam-like residue detector was a DSSSD with a thickness of 500 µm, an active area of 122×44 mm2, 122 vertical strips and 40 horizontal strips. This detector was placed at about 13.4 mm from the beam axis. With this distance we ensured that the rate of elastic scattered beam ions over the whole detector was well within the expected radiation-damage tolerance range of the detector, which remained operational during the full experiment. This detector was also placed inside a pocket and separated from the UHV by a thin stainless-steel window of 25 mm. This pocket belongs to GSI and was in a movable drive that moved the detector in and out to protect it from a possible impact with the beam after injection. The detector was used in air. Fig. 5 shows a picture of the beam-like residue detector system.
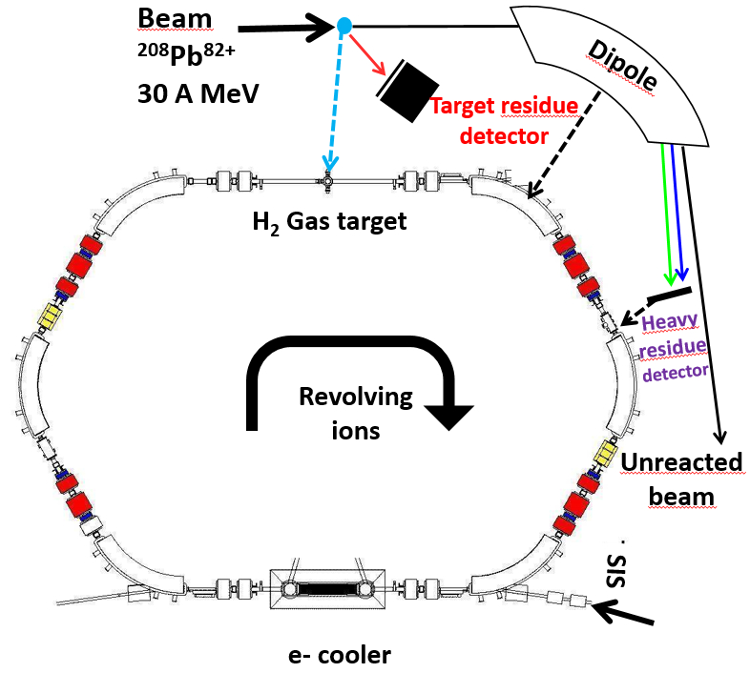
Fig. 3: The lower part shows a schematic view of the ESR. The upper part shows the setup for the proof of principle experiment. The trajectories of the heavy beam-like residues produced after emission of g rays (82+Pb208) and a neutron (207Pb82+) are shown in blue and green, respectively.
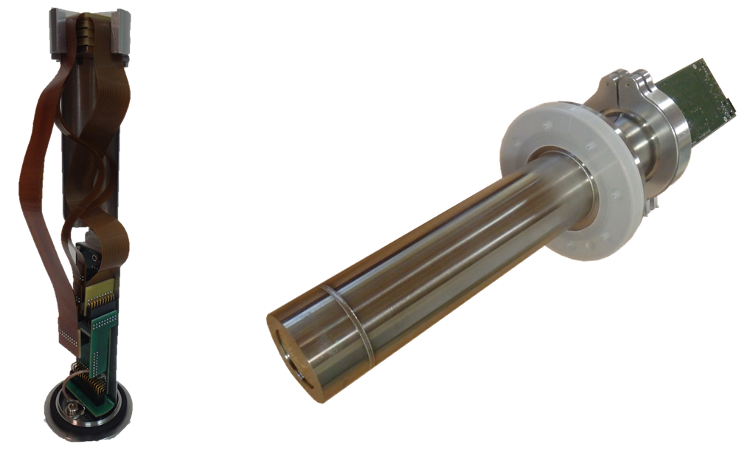
Fig. 4: Pictures of the telescope (left) and of the pocket that houses it (right).
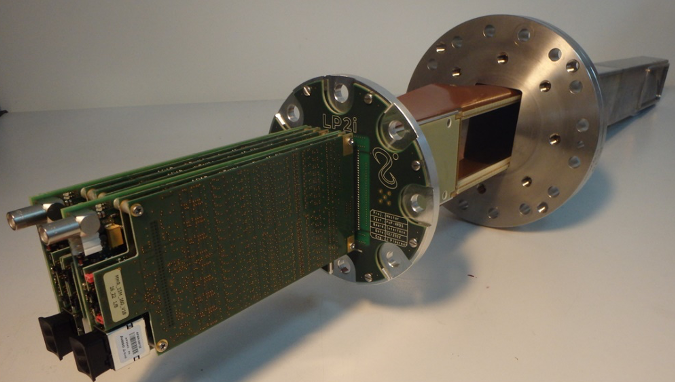
Fig 5: Picture of the beam-like residue detection system as being inserted in its pocket.
This first NECTAR experiment will allow us to measure for the first time the probability that the excited 208Pb decays by emitting gamma-rays or a neutron. This is very complicated with usual methods as it requires detecting gamma rays and neutrons, for which the detection efficiencies are very low. With NECTAR we have taken advantage of the unique capability of the ESR storage ring to separate the residues of 208Pb formed after gamma-ray (208Pb) and neutron emission (207Pb). We have then been able to extricate these residues from the huge background created by the elastic scattering of the beam by detecting the beam residues in coincidence with the scattered protons. Figure 6 shows the position spectra of heavy residues detected without (left) and with (right) the coincidence with the protons. The heavy residues of the projectile produced after its de-excitation can only be seen in the coincidence spectrum: 208Pb nuclei that de-excite by gamma emission are in the left bump, while 207Pb nuclei produced after neutron emission are located in the right bump. We can see a very clear separation of the two bumps. In addition, the beam residues were detected with efficiencies close to 100%.
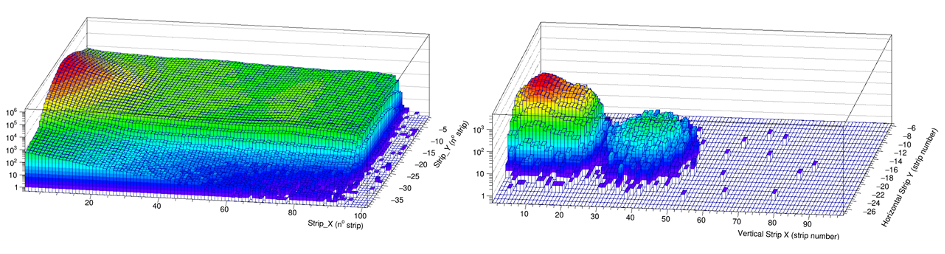
Fig 5: Picture of the beam-like residue detection system as being inserted in its pocket.
We can then conclude that this first NECTAR experiment was a real success as it has allowed us to validate the detection systems for target and beam residues, the data acquisition system and the methodology to determine very accurate gamma and neutron-emission probabilities with NECTAR. The collected data are being currently analyzed.
COLLABORATIONS
Presently our main collaborators are:
- GSI, Darmstadt, Germany
- Max-Planck Institute for Nuclear Physics, Heidelberg, Germany
- Goethe Universität Frankfurt, Frankfurt am Main, Germany
- CEA, DAM, DIF, France
Outreach
- Interview with Beatriz Jurado: https://in2p3.cnrs.fr/fr/cnrsinfo/beatriz-jurado-laureate-de-lerc-advanced
- Video available on youtube: https://www.youtube.com/watch?v=UhhyoEj96bQ
PhD thesis
PhD Thesis of Michele Sguazzin, defended on the 28 March 2023 at the University of Bordeaux
The thesis manuscript can be downloaded here: https://theses.hal.science/tel-04192424«
PUBLICATIONS
On the method :
- First measurement of the neutron-emission probability with a surrogate reaction in inverse kinematics at a heavy-ion storage ring »
M. Sguazzin et al., Submitted to PRL, http://arxiv.org/abs/2312.13742 - Indirect measurements of neutron-induced reaction cross sections at heavy-ion storage rings
M. Sguazzin et al., EPJ Web of Conferences 284, 01008 (2023) - Indirect measurements of neutron-induced reaction cross sections at storage rings
M. Sguazzin et al., EPJ Web of Conferences 279, 11006 (2023) - Determining neutron-induced reaction cross sections through surrogate reactions at storage rings
M. Sguazzin et al., 2023 J. Phys.: Conf. Ser. 2586 012082 - Surrogate Reactions at Heavy-Ion Storage Rings: The NECTAR Project
Beatriz Jurado, (2023) Nuclear Physics News, 33:3, 19-22, DOI: 10.1080/10619127.2023.2230849 - Pérez Sànchez et al., Phys. Rev. Lett. 125, 122502 (2020).
- Henriques et al., J. Phys.: Conf. Ser. 1668 012019 (2020). (Open access): https://iopscience.iop.org/article/10.1088/1742-6596/1668/1/012019/meta
- Jurado et al., JPS Conf. Proc. 35, 011001 (2021), https://doi.org/10.7566/JPSCP.35.011001
About R&D work on solar cell detectors :
- Henriques et al, Nucl. Instrum. Methods A 969, 16394 (2020).
Talks & Posters
- Invited seminar at the IJCLab, 22 March 2024, Orsay, France
Sguazzin, “Study of surrogate reactions at heavy-ion storage rings” - British-German WE-Heraeus-Seminar, “Nuclear Astrophysics with Ion Storage Rings”, 29 Jan – 02 Feb 2024, Bad Honnef, Germany
Wloch, “Detector developments and technical aspects of the second NECTAR experiment” - British-German WE-Heraeus-Seminar, “Nuclear Astrophysics with Ion Storage Rings”, 29 Jan – 02 Feb 2024, Bad Honnef, Germany
Berthelot, “Detailed simulations for the next surrogate reaction experiment at the ESR storage ring” - British-German WE-Heraeus-Seminar, “Nuclear Astrophysics with Ion Storage Rings”, 29 Jan – 02 Feb 2024, Bad Honnef, Germany
Sguazzin, “First NECTAR experiment and future use of solar cells as heavy-ion detectors in storage rings” - British-German WE-Heraeus-Seminar, “Nuclear Astrophysics with Ion Storage Rings”, 29 Jan – 02 Feb 2024, Bad Honnef, Germany
Jurado, invited, “Surrogate reactions at heavy-ion storage rings” - Journées R&T de l’IN2P3, 6-8 November 2023, Strasbourg, France
Jurado, invited, “Nuclear rEaCTions At storage Rings, NECTAR” - Nuclear rEaCTions At storage Rings, NECTAR B. Jurado, invited
- 50 International meeting on fundamental physics and XV CPAN days, 2-6 October 2023, Santander, Spain
B. Jurado, Invited plenary talk, “Nuclear reactions at heavy-ion storage rings” - Mazurian Lakes conference on Physics, 3-9 September 2023, Piaski, Poland
B. Jurado, Invited talk, “Surrogate reactions in inverse kinematics at heavy-ion storage rings” - The ELEMENTS annual conference, 11-13 July 2023, Bad Nauheim, Germany
B. Jurado, Invited talk, “Surrogate reactions at heavy-ion storage rings” - Gordon Research Conference in Nuclear Chemistry 11-16 June 2023, New London, USA,
B. Jurado, Invited talk, “Indirect measurements of neutron-induced reaction cross sections at heavy-ion storage rings” - Invited seminar at the Max-Planck Institute of Nuclear Physics in Heidelberg, 25/05/2023
M. Sguazzin, “Surrogate reactions at heavy-ion storage rings” - ARIS 2023, 4-9 Juin 2023, Avignon, France
M. Sguazzin, oral contribution, “Indirect measurements of neutron-induced reaction cross sections at heavy-ion storage rings” - Symposium on precision physics with highly charged ions held during the spring meeting of the German Physical Society, 5-10 March 2023, Hannover, Germany
B. Jurado, Invited talk, “Indirect measurements of neutron-induced reaction cross-sections at heavy-ion storage rings” - Invited webinar of the GDR RESANET, 12 December 2022,
B. Jurado, « Nuclear Reactions at storage rings »
https://www.youtube.com/channel/UCbQnzKbKdlIQrSv6kXa5n_A - Invited Webinar of the Nuclear Physics Division of the University of Warsaw, 17th November 2022
B. Jurado, “Indirect measurements of neutron-induced reaction cross sections at storage rings” - European Nuclear Physics Conference, EuNPC 2022, 20-28 October 2022, Santiago de Compostela, Spain
B. Jurado, Invited plenary talk, “Nuclear fission at storage rings - Assemblée générale du GDR RESANET, 26-27 Septembre 2022, Lyon, France
M. Sguazzin, invited oral, « Indirect measurements of neutron-induced reaction cross sections at storage rings” - 28th International Nuclear Physics Conference (INPC 2022)”, 11-16 September, Cape Town, South Africa
J. A. Swartz, “Determining neutron-induced reaction cross sections through surrogate reactions at storage rings”
- 19th SPARC Topical Workshop, 6-9 September, 2022, Hybrid
J. A. Swartz, “Determining neutron-induced reaction cross sections through surrogate reactions at storage rings” - Nuclear Physics in Astrophysics-X, NPA-X, 4-9 September 2022, CERN, Switzerland
B. Jurado, “Indirect measurements of neutron-induced reaction cross sections at storage rings” - 15th International Conference on Nuclear Data for Science and Technology, ND2022, 24-28 July, 2022, Online.
B. Jurado, Invited, “Indirect measurements of neutron-induced reaction cross sections at storage rings” - Invited Webinar of the Nuclear Physics Division of the University of Warsaw, 17th November 2022
B. Jurado, “Indirect measurements of neutron-induced reaction cross sections at storage rings” - SNAQ (School on Nuclear Astrophysics Questions), 12 January 2022, Jacobus Swartz, oral contribution, «
- Ecole Thématique PhyNuBE : Première rencontre de Physique Nucléaire de Basse Energie 2021, 5–10 déc. 2021 M. Sguazzin, Oral contribution, « Response of solar cells to heavy ions at energies close to 10 AMeV »
- Ecole Thématique PhyNuBE : Première rencontre de Physique Nucléaire de Basse Energie 2021, 5–10 déc. 2021 J. Swartz, Oral contribution, « Determining neutron-induced reaction cross sections through surrogate reactions at storage rings »
- Junior Researcher Webinar of the GDR RESANET, 29 November 2021 M. Sguazzin “The NECTAR project and solar cell studies”
- Journée scientifique du département Sciences de la Matière et du Rayonnement, 12 November 2021, Bordeaux Jurado, invited, «Nuclear reactions at heavy-ion storage rings”
- Colloque GANIL, 26 September-1st October 2021, Autrans-Meudre en Vercors, France Sguazzin, “Investigation of the response of solar cells to heavy ions at energies close to 10 A MeV”
- Colloque GANIL, 26 September-1st October 2021, Autrans-Meudre en Vercors, France Jurado, “Indirect measurements of neutron-induced cross sections at storage rings”
- 6th International Symposium on Nuclei in the Cosmos, Chengdu, China (September 21-25, 2021), online A. Swartz, Poster « Determining neutron-induced reaction cross sections through surrogate reactions at storage rings »
- 18th SPARC topical workshop, 6-9 September 2021, online Swartz, poster, “Proof of principle experiment at the ESR for the NECTAR project”
- North American Storage Rings and Neutron Captures Workshop, 28-30/06/2021, online Jurado, invited talk, “Surrogate reactions at storage rings”
- FAIR-GSI Joint Scientific Council, 18/06/2021, online Jurado, invited talk, NECTAR “Nuclear Reactions at Storage Rings”